By Mahshid Ahmadi, University of Tennessee
Many human activities release pollutants into the air, water and soil. These harmful chemicals threaten the health of both people and the ecosystem. According to the World Health Organization, air pollution causes an estimated 4.2 million deaths annually.
Scientists are looking into solutions, and one potential avenue is a class of materials called photocatalysts. When triggered by light, these materials undergo chemical reactions that initial studies have shown can break down common toxic pollutants.
I am a materials science and engineering researcher at the University of Tennessee. With the help of robots and artificial intelligence, my colleagues and I are making and testing new photocatalysts with the goal of mitigating air pollution.
Breaking down pollutants
The photocatalysts work by generating charged carriers in the presence of light. These charged carriers are tiny particles that can move around and cause chemical reactions. When they come into contact with water and oxygen in the environment, they produce substances called reactive oxygen species. These highly active reactive oxygen species can bond to parts of the pollutants and then either decompose the pollutants or turn them into harmless – or even useful – products.
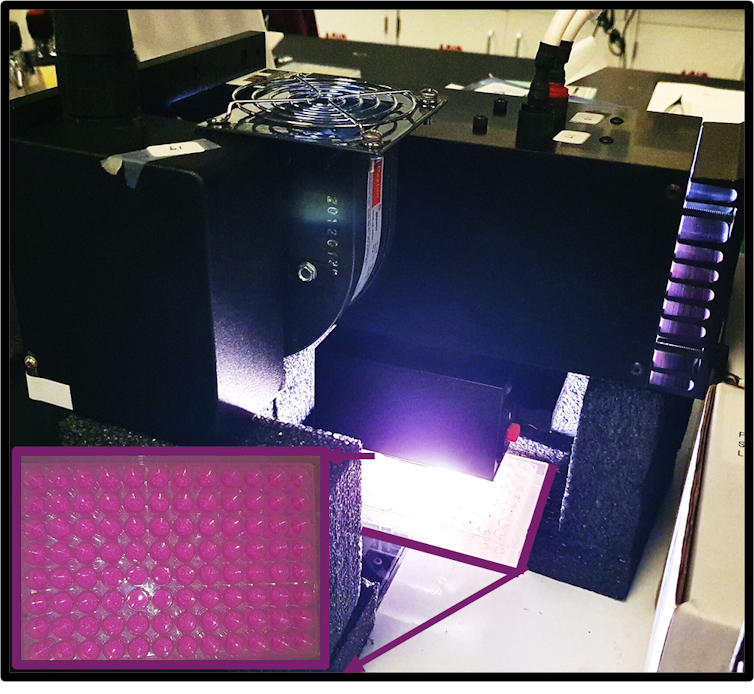
Astita Dubey
But some materials used in the photocatalytic process have limitations. For example, they can’t start the reaction unless the light has enough energy – infrared rays with lower energy light, or visible light, won’t trigger the reaction.
Another problem is that the charged particles involved in the reaction can recombine too quickly, which means they join back together before finishing the job. In these cases, the pollutants either do not decompose completely or the process takes a long time to accomplish.
Additionally, the surface of these photocatalysts can sometimes change during or after the photocatalytic reaction, which affects how they work and how efficient they are.
To overcome these limitations, scientists on my team are trying to develop new photocatalytic materials that work efficiently to break down pollutants. We also focus on making sure these materials are nontoxic so that our pollution-cleaning materials aren’t causing further pollution.
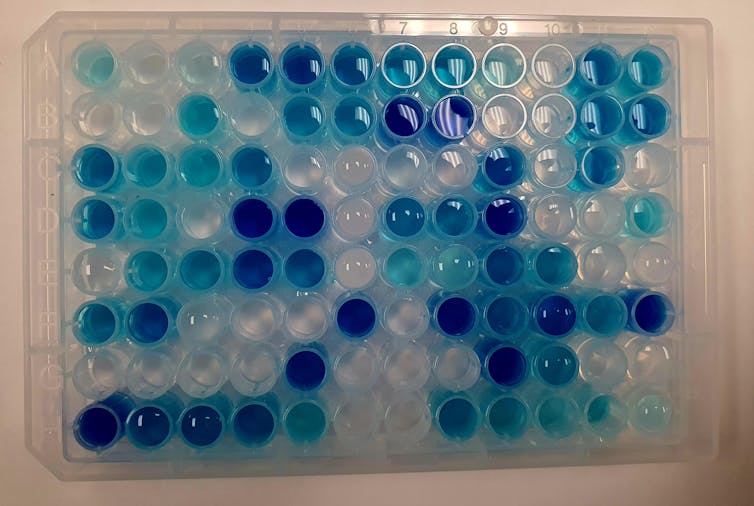
Astita Dubey
Teeny tiny crystals
Scientists on my team use automated experimentation and artificial intelligence to figure out which photocatalytic materials could be the best candidates to quickly break down pollutants. We’re making and testing materials called hybrid perovskites, which are tiny crystals – they’re about a 10th the thickness of a strand of hair.
These nanocrystals are made of a blend of organic (carbon-based) and inorganic (non-carbon-based) components.
They have a few unique qualities, like their excellent light-absorbing properties, which come from how they’re structured at the atomic level. They’re tiny, but mighty. Optically, they’re amazing too – they interact with light in fascinating ways to generate a large number of tiny charge carriers and trigger photocatalytic reactions.
These materials efficiently transport electrical charges, which allows them to transport light energy and drive the chemical reactions. They’re also used to make solar panels more efficient and in LED lights, which create the vibrant displays you see on TV screens.
There are thousands of potential types of hybrid nanocrystals. So, my team wanted to figure out how to make and test as many as we can quickly, to see which are the best candidates for cleaning up toxic pollutants.
Bringing in robots
Instead of making and testing samples by hand – which takes weeks or months – we’re using smart robots, which can produce and test at least 100 different materials within an hour. These small liquid-handling robots can precisely move, mix and transfer tiny amounts of liquid from one place to another. They’re controlled by a computer that guides their acceleration and accuracy.
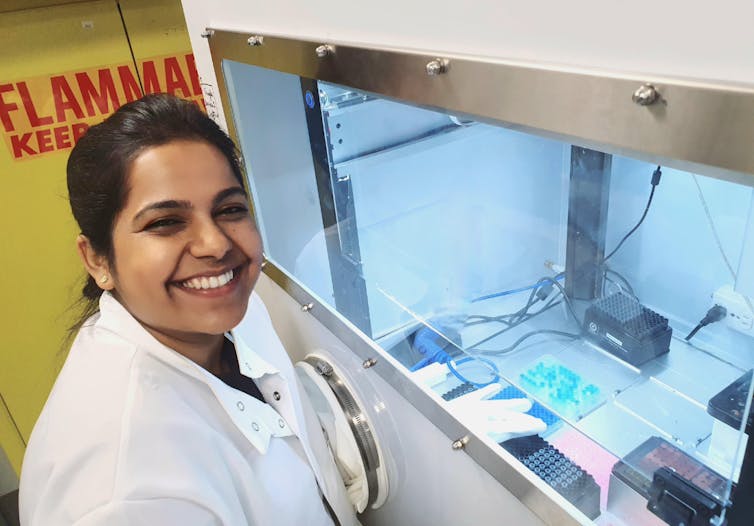
Jordan Marshall
We also use machine learning to guide this process. Machine learning algorithms can analyze test data quickly and then learn from that data for the next set of experiments executed by the robots. These machine learning algorithms can quickly identify patterns and insights in collected data that would normally take much longer for a human eye to catch.
Our approach aims to simplify and better understand complex photocatalytic systems, helping to create new strategies and materials. By using automated experimentation guided by machine learning, we can now make these systems easier to analyze and interpret, overcoming challenges that were difficult with traditional methods.
About the Author:
Mahshid Ahmadi, Assistant Professor of Materials Science and Engineering, University of Tennessee
This article is republished from The Conversation under a Creative Commons license. Read the original article.